As the name suggests, antiwear additives help to prevent wear in one way or another. However, what makes them unique compared to other additives in lubricants? Why are they used more predominantly in specific applications than other applications? This article explores antiwear additives, why they are needed, and how they work.
What Are Antiwear Additives?
According to (Bloch, 2009), antiwear agents can also be called mild EP (Extreme Pressure) additives. In some cases, they may also act as antioxidant additives (depending on their chemical structure). In essence, antiwear additives protect against friction and wear when the surfaces experience moderate boundary conditions.
During moderate boundary conditions, the full film of the lubricant has not yet formed, and asperities on both surfaces can come into contact with each other. As such, antiwear additives can also be called boundary lubrication additives.
Usually, these antiwear additives react chemically with the metal to form a protective layer. This layer or coating will allow the two surfaces to slide over each other with low friction and minimal metal loss. As such, antiwear additives have also adopted the nickname “anti-scuff” additives.
According to Pirro, Webster, & Daschner, 2016, the adsorbed film on metal surfaces is formed from long-chain materials. In these cases, the polar ends of the molecules attach to the metal while the projecting ends of the molecules remain between the surfaces.
Under mild sliding conditions, wear is reduced; however, under severe conditions, molecules can be rubbed off such that the wear-reducing effect is lost. When this happens, it is evident in the oil analysis data with the presence of wear metals in large quantities.
In essence, antiwear additives help protect the oil while reducing friction, protecting the surfaces, and, in some cases, enhancing the oil to be more resistant to oxidation. While they can perform these functions, it must be noted that there are many different types of antiwear additives.
Types Of Antiwear Additives and How They Work
There are many types of antiwear additives, but they typically all fall under the category of polar materials such as fatty oils, acids, and esters, according to Pirro, Webster & Daschner, 2016. According to Mortier, Fox, & Orszulik, 2010, several compounds can form surface films to help protect against friction and wear.
These include:
- Oxygen-containing organic compounds (with a polar head that can adsorb to surfaces). These can include alcohols, esters, and carboxylic acids.
- Organic compounds containing nitrogen groups
- Organic sulphur compounds which can form reacted films at surfaces
- Organic phosphorus compounds which can form reacted films at surfaces
- Organic boron compounds which may form reacted films at surfaces
- Organic molybdenum compounds which can form MoS2 film on surfaces
- ZDDPs, which can form polymeric films on surfaces
While this is an exhaustive list, the more popular ones are listed below. In this next part of the article, we will also dive into how they function.
Organic Oxygen Compounds
According to Mortier, Fox, & Orszulik, 2010, these compounds usually include esters, alcohols and acids. These are generally responsible for improving the “oiliness” or reducing the friction for most lubricants. However, how does this work?
Carboxylic acids form metallic soaps with the contacting surfaces. According to Mortier, Fox, & Orszulik, 2010, some evidence suggests that the upper limit of friction coincides with the melting point of the metal soap. As such, when the upper limit of friction is reached, the metallic soap melts, protecting the surface and performing its antiwear function.
Interestingly, there has been a debate concerning whether these long-chain surfactant friction modifiers reduce friction by forming adsorbed films of monolayer thickness or if they form thick films equivalent to several or many multilayers.
Again, as per Mortier, Fox, & Orszulik, 2010, after experimenting, it was concluded that some of these types of additives form thick boundary films while others do not.
The thick boundary films result from the formation of insoluble iron (II) oleate on the rubbing surfaces. For metal oleates, this will only occur for metals lower than iron in the electrochemical series.
Thus, when speaking about organic oxygen compounds, they help to reduce the friction in lubricants by forming layers on the contacting surfaces.
Organophosphorus Esters
These types of esters have long been used as antiwear additives, according to Mortier, Fox, & Orszulik, 2010. There are two different types of reaction films which are typically formed:
- Films derived from tricresyl phosphate which form thin films (0.1-2nm) consisting of low shear strength FePO4 and FePo4.2H2O
- Films consisting of iron (III) monoalkyl/aryl phosphate oligomers are thicker (approximately 100-300nm) and polymeric.
It is important to note that for the tricresyl phosphate (TCP) to be effective, the presence of oxygen, water, and other polar impurities is necessary to form the reaction film. Typically, the hydrolysis of the ester occurs initially, which releases phosphoric acid. This is then critical in the formation of the surface oxide film.
Another noteworthy function of the ester of phosphoric acid is that it helps ensure the solubility of the product in the oil. It can also aid in rust protection by hydrolysis to the phosphoric acid.
During the formation of the film, there is a loss of an alkyl group by hydrolysis, which generates two P-O ligands for coordination. This phosphate anion, which was formed, has reduced oil solubility, which allows for the boundary layer of oil covering the metal surface.
Eventually, as the polymer continues growing, the film moves from a soft, viscous liquid to that of a glass-like solid. This glass-like solid allows the surfaces to stay separated, thus reducing wear.
Essentially, organophosphorus esters form films that can either be very thin or thicker and glass-like, depending on their nature. While they act as antiwear additives, they can also perform the function of rust inhibition in the appropriate environments.
Molybdenum Sulfur
Coyle et al., Patent No. 4,995,996, 1991 recognize Molybdenum disulphide as a lubricant additive and discuss its origins. They mention that molybdic xanthine typically decomposes under particular conditions to form the molybdenum sulfide on protected materials. The use of thiosulfenyl xanthates has also been formulated for particular ashless lubricants.
As per Mortier, Fox, & Orszulik, 2010, compounds such as MoDTC (molybdenum dithiocarbamate) or MoDDP (molybdenum dithiophosphate) typically react with the surfaces to produce the famous molybdenum disulphide. In this compound, there is an ease of shearing, which leads to unusually low coefficients of friction.
A synergistic relationship exists between MoDTC and ZDDP. While MoDTC does not form low friction layers independently, these layers are only formed when ZDDP is present. The layer of MoS2 is only formed on top of the glass of ZDDP reaction products. The ZDDP layer acts as a source of sulphur, reduces the oxidation of MoS2 and limits the diffusion of sulphur from MoS2 into the ferrous substrate.
Interestingly, Molybdenum disulphide (also commonly known as “Moly”) is extremely popular in grease applications especially in the mining industry. “Moly” is known for being a solid additive to grease thickeners for specific applications.
As seen above, it may not exactly be “Moly” added to the lubricant, but rather, it is only created when its parent compound decomposes and is formed.
Zinc Dialkyldithiophosphates (ZDDP)
These are the most commonly used antiwear additives on the market and are known by their chemical abbreviation ZDDP. Originally, ZDDP was developed as an antioxidant additive. However, it has been used in many applications, such as engine, hydraulic, and even circulating oils, as both an antiwear and antioxidant additive.
According to Bruce, 2012, The Ecole Centrale de Lyon / Shell Corporation collaboration made significant conclusions on ZDDP performance. This study shows that ZDDP produces a thin film of iron sulfide and zinc sulfide nearest to the metal surface. Next, there is a zinc polyphosphate layer, made up of long-chain zinc polyphosphates and then soluble alkylphosphates, closest to the oil layer.
According to Zhang & Spikes, 2016, at very high temperatures (above 150°C), ZDDP reacts slowly to form films on solid surfaces. This occurs despite the absence of rubbing and is called “thermal films .” However, at lower temperatures (below 25°C) in the presence of rubbing films in a ZDDP lubricant, these ZDDP films are generated more rapidly. These are called “tribofilms”. Based on analysis, it is suggested that both films have similar structures.
It has also been shown (through inelastic electron tunneling spectroscopy, IETS with Yamaguchi and Ryason) that secondary ZDDP is adsorbed much more readily than primary ZDDP. On the other hand, alkaryl ZDDP is hydrolyzed on adsorption onto aluminum oxide surfaces.
According to Mortier, Fox, & Orszulik, 2010, ZDDP reduces wear by forming relatively thick boundary lubrication films. These are usually 50-150nm thick and are based on a complex glass-like structure (as mentioned earlier). The figure below, taken from Mortier, Fox, & Orszulik, 2010, shows the structure of this ZDDP glass film.
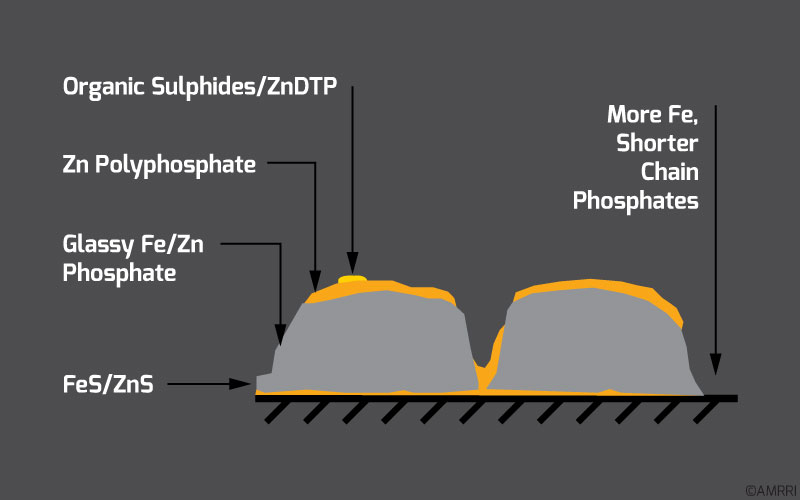
Structure and composition of a ZDDP glass film (taken from Mortier, Fox, & Orszulik, 2010)
The strength of the ZDDP’s antiwear function lies in the structure of the alkyl groups. Chain branching and chain length have critical roles in this determination. Short-chain primary alkyl groups are more reactive than long primary alkyl groups.
As Mortier, Fox, & Orszulik, 2010, explain, the ZDDPs most efficient at antiwear film formation typically suffer depletion due to thermal effects. Under very high temperatures and/or long drain service, the most active ZDDP may not provide the best wear protection.
The Difference Between Antiwear Additives and Extreme Pressure Additives
The terms antiwear additives and extreme pressure additives are often used interchangeably, suggesting that they provide the same functions in a lubricant. This is not exactly true. While there are many similarities in how they function, both additives have distinct functions in protecting lubricants.
Both are film-forming additives (Bruce, 2012). Their functions are to reduce wear between two contacting surfaces or reduce friction to lower the heat produced between the two rubbing surfaces.
They can also be classified as boundary additives that can be temperature-dependent (EP additives) or non-temperature-dependent (Antiwear additives). They both function to mitigate against wear, which is usually caused during boundary lubrication where higher speeds, loads, or temperatures can cause contact with the asperities.
One of the significant differences, as noted by Mang & Dresel, 2007 is that antiwear additives are designed to reduce wear when the system is exposed to moderate stress. On the other hand, EP additives are much more reactive. These are used when the system’s stress is very high to prevent the welding of moving parts.
According to (Bruce, 2012), there are four main groups of commercially available EP additives based on the structures containing phosphorus, sulphur, chlorine, and overbased sulfonates. He explains that the phosphorus, sulphur, and chlorine-containing EP additives are activated by heat over a range of temperatures.
For instance, chlorine-containing EP additives are usually activated between 180-240°C, phosphorus-containing additives are activated at higher temperatures, and sulphur-containing additives operate at 600-1,000°C.
On the other hand, overbased sulfonates contain a colloidal carbonate that reacts with iron to form a thin-film barrier layer between tribocontacts. This protects the surface from direct contact and welding.
As we can see, antiwear and EP additives protect the surfaces between which the lubricant exists. However, they are activated differently and subsequently perform two different functions.
Antiwear additives protect against wear and are not temperature dependent, while EP additives are activated by high stress to prevent the welding of moving parts.
Both functions are essential to protecting the system from additional wear and ensuring it remains operational.
References
Bloch, H. (2009). Practical lubrication for industrial facilities, Second edition. Lilburn: Fairmont Press Inc.
Bruce, R. W. (2012). Handbook of Lubrication and Tribology, Volume II, Theory and Design, Second Edition. Boca Raton: CRC Press Taylor and Francis Group.
Coyle, C. L., Greaney, M. A., Stiefel, E. I., Francis, J. N., & Beltzer, M. (1991, Feb 26). United States of America Patent No. 4,995,996.
Mang, T., & Dresel, W. (2007). Lubricants and Lubrication, Second, Completely Revised and Extended Edition. Weinheim: WILEY-VCH Verlag GmbH & Co. KGaA.
Mortier, R. M., Fox, M. F., & Orszulik, S. T. (2010). Chemistry and Technology of Lubricants, Third Edition. (C. Bovington, Ed.) Dordrecht Heidelberg: Springer Science+Business Media B.V. doi:10.1023/b105569_3
Pirro, D. M., Webster, M., & Daschner, E. (2016). ExxonMobil, Lubrication Fundamentals, Third Edition, Revised and Explained. USA: CRC Press Taylor and Francis Group.
Zhang, J., & Spikes, H. (2016). On the Mechanism of ZDDP Antiwear Film Formation. Tribol Lett, pp. 1–2.