Why Water in Oil Is So Dangerous
From an early age, we learn that oil and water do not mix. While that is not exactly true in the world of lubrication, it is true to say that any degree of water in a lubricant can cause irreparable harm to both the lubricated components and the oil.
When water enters an oil sump or reservoir, it exists in one of three distinct phases: free water, emulsified water, or dissolved water. Each poses different challenges for lubricating oils, but all can significantly reduce the life of the equipment, resulting in excessive maintenance costs and unscheduled downtime.
How Water Exists in Oil: Dissolved, Emulsified, and Free
As the name implies, dissolved water means that the water and oil mix to form a solution, just like dissolving salt in warm water. While this may seem counterintuitive, since water is polar while oil is largely non-polar, the presence of polar components, such as oil additives, degradation by-products, or certain contaminants, can cause oil and water to mix.
The amount of water that can be dissolved in a new oil is dependent on the type of base oil, the amount and type of additives contained in the oil, and the temperature.
For mineral oils or hydrocarbon-based synthetic oils, the base oil’s affinity for water is very low, meaning little water will dissolve in the oil. However, due to the polar nature of some base oils, particularly API Group V base oils, significant quantities of water can be dissolved in the oil.
Likewise, additives affect the solubility of water in oil. While lightly additized oils, such as turbine oils, contain less than 5% additives and therefore cannot help water become soluble in the oil, more heavily additized oils, such as gear oils, hydraulic fluids, and engine oil, can hold much higher concentrations of water in the dissolved phase.
In fact, a heavy-duty engine oil that contains as much as 30% by volume of additives can hold more than 2000 ppm (0.2% v/v) of water in oil.
Additives and temperature can make oil hold far more water than you expect.
Similarly, temperature affects the amount of water that can be dissolved in oil. At room temperature, a conventional R&O oil may hold up to 120-150 ppm (0.012-0/015% v/v) of water in solution. However, as the oil cools, the solubility of water in oil decreases to the point where at 40°F, the oil may only hold 20-40 ppm of water in solution (Figure 1).
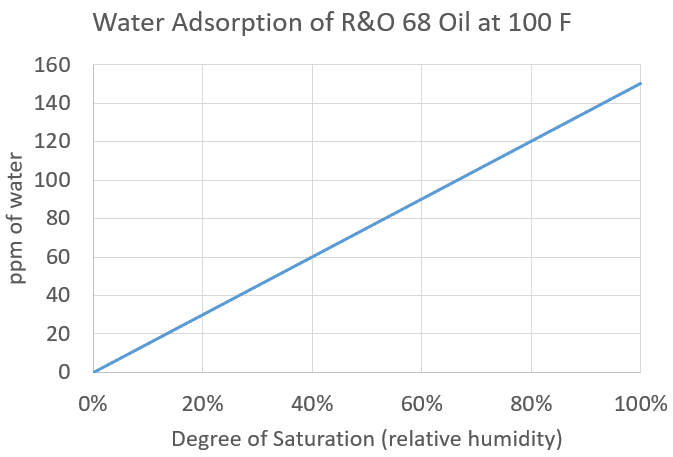
Figure 1: Solubility of R&O 68 at 100°F
The maximum amount of water that an oil can hold in solution at a given temperature is referred to as the saturation point. Much like the dew point of air, which is the temperature at which the air becomes saturated with moisture and starts to precipitate water in the form of fog or dew, the saturation point of the oil is the point where no more water can be dissolved in the oil, forcing any additional moisture to come out of solution.
When water comes out of solution, it will coexist with the oil in one of two phases: free or emulsified. Free water refers to water that has completely separated from the oil and settled to the bottom of the tank or oil sump. By contrast, emulsified oil refers to a suspension of small water droplets (the dispersed phase) in the oil (the continuous phase).
Whether mixed oil and water exist in the free or emulsified phase depends largely on a property of the oil known as demulsibility. The demulsibility of a lubricant can be defined as the ease with which water and oil separate. If an oil has a high degree of demulsibility, it will very rapidly shed water into the free phase (Figure 2). By contrast, poor or low demulsibility means that the water will remain mixed with the oil, resulting in a cloudy or hazy appearance (Figure 3).
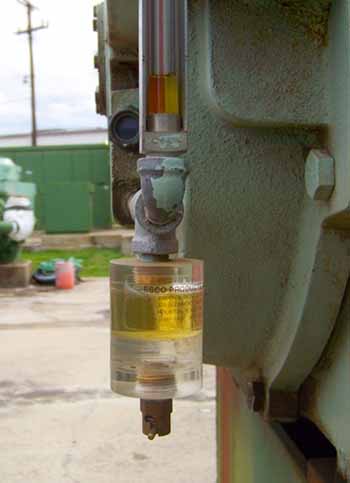
Figure 2: Demulsified oil and water in a Bottom Sediment & Water (BS&W) bowl
Like solubility, demulsibility is dependent on the type of oil, the degree of oil degradation, and the presence of specific contaminants. Light additive oils will separate (demulsify) quickly, typically within 5-10 minutes. However, more heavily additized and severely degraded oils will take much longer to separate.
Some oils, particularly those that contain detergents, are severely degraded or have become contaminated with certain contaminants, such as soaps or other process fluids, may lose the ability to shed water completely, forming a stable emulsion (Figure 3).
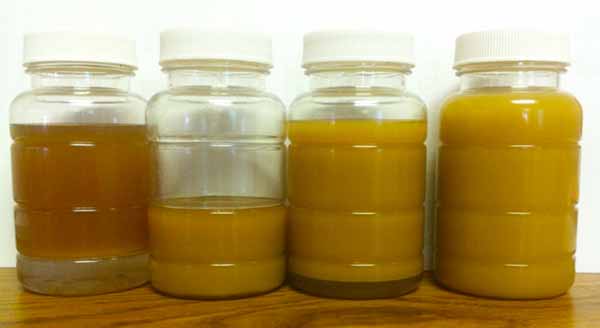
Figure 3: When oil becomes contaminated with process chemicals, it often loses its demulsibility
When it comes to a lubricant’s ability to perform its job, the presence of free or emulsified water is of greatest concern. This is particularly true for wet-sump applications, such as small process pumps or splash-lubricated gearboxes, since the lubricated components operate directly in the oil sump.
Free and emulsified water in wet-sump applications not only causes rust and corrosion to occur but can also significantly affect film strength, leading to an increase in the rate of oil degradation and the formation of sludge and varnish.
Moisture leads to rust, weak film strength, and rapid oil degradation.
Figure 4 shows the impact that water can have on equipment life. In this seminal study, researchers at Timken Bearing were able to derive an empirical relationship between the amount of water in an oil (specifically, an R&O ISO VG 68 fluid) and the life expectancy of a rolling-element bearing.
This would mirror exactly what might happen in a small centrifugal pump. As the graph illustrates, bearings that operate with water above the saturation point (approximately 100-150 ppm in this case) will have a significantly reduced life expectancy, often as low as 50% of the bearing’s anticipated life.
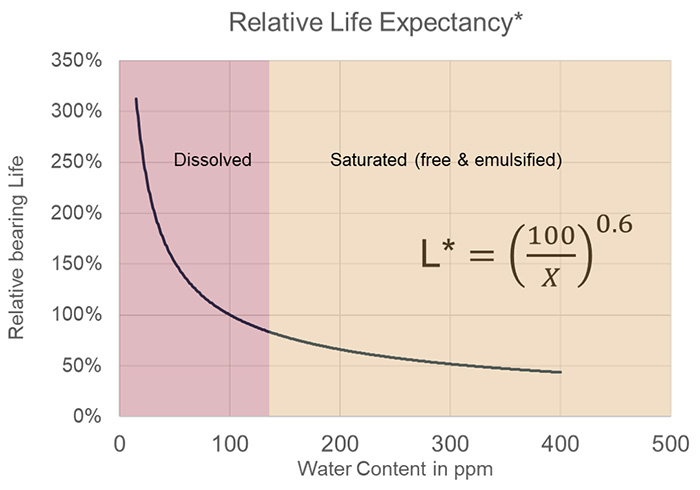
Figure 4: Impact of Water on Rolling Element Bearing Life (Ref: R. E. Cantley, ASLE Transactions Vol. 20. 3. 244-248, 1977)
Preventing Moisture Ingress: Techniques That Work
In process industries such as pulp and paper, petrochemical refining, food and beverage, and wastewater treatment, water is a constant presence, as are pumps and gearboxes. Because of this, progressive companies seek to control water using improved seals, pre-filtration of new oils, desiccant breathers, and specialized filtration such as super-absorbent polymers and vacuum dehydrators. Each of these can be effective, but no one method alone can solve all the potential problems.
Water can enter an oil in several ways, but one of the most common is when equipment operates in a high-humidity environment, either inside or outside. Even with the highest degree of care and attention, humid air can still enter equipment if it is not properly protected.
When water and oil coexist, the degree of saturation of the oil always matches the relative humidity of the air above the oil, and vice versa. So, if the relative humidity of the air is 80%, the oil in contact with the air will be 80% saturated, assuming the oil and air are at the same temperature. This is due to Henry’s Law, first discovered in 1803, which states:
“At a constant temperature, the amount of a given gas that dissolves in a given type and volume of liquid is directly proportional to the partial pressure of that gas in equilibrium with that liquid.”
While this concept may seem somewhat abstract based on its definition, Henry’s Law explains several everyday observations, such as why the US Gulf Coast is so humid or why soda goes flat relatively quickly after the can is opened.
In the case of soda, when the can is sealed at the bottling plant, a small gap, known as the headspace, is left at the top of the can. Since the soda is saturated with carbon dioxide, the headspace inside the can is also saturated with carbon dioxide due to Henry’s Law.
However, once the can is opened, the headspace becomes the entire atmosphere. Recognizing this imbalance between CO2 content in the air versus the soda, the soda will “fizz” until the concentration of CO2 in the soda matches the (low) concentration of CO2 in the air.
Why Headspace Humidity Control Is Essential
In oil, the same holds. If oil is in contact with water vapor due to high humidity, it will become saturated very quickly. However, if the headspace humidity is lowered, for example through the use of a desiccant breather, the oil will yield water due to Henry’s Law, effectively dehydrating the oil (Figure 5).
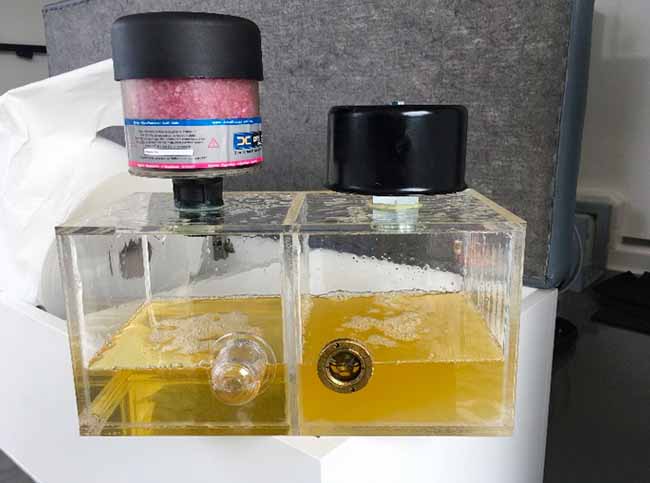
Figure 5: When the air above the oil is maintained at very low humidity, the oil will also maintain a low level of water saturation
In industrial equipment, a similar effect can be observed. Figure 6 shows the impact of headspace humidity on two identical centrifugal pumps operating outside in relatively high humidity (74%) and high temperature (93°F). Both pumps were filled with pre-filtered oil to remove as much moisture as possible and were equipped with bearing isolators to prevent the migration of contaminants through the shaft-seal interface.
In addition, both pumps were completely sealed from the outside environment by plugging the breather/fill port; one was sealed with a simple pipe plug, and the second was sealed with a “smart” desiccant breather equipped with a humidity sensor.
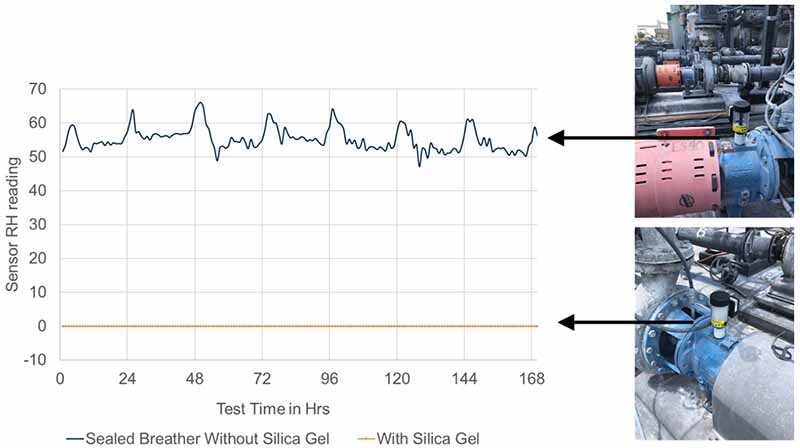
Figure 6: Comparison of two identical process pumps, with and without desiccant breather headspace protection
As shown in Figure 6, the pump without headspace humidity protection exhibited relatively high internal humidity, which fluctuated between 55% and 65% due to daily temperature fluctuations. Despite being nominally sealed, humid air exchange is occurring between the outside and inside, likely through the bearing isolator.
By contrast, the pump with headspace protection maintained a very low internal humidity due to the silica gel actively dehumidifying the headspace. Based on the graph shown in Figure 4, it can be anticipated that the bearing life of the pump with dry headspace would be 20-40% longer than that of the pump with no protection, indicating that both bearing isolators and desiccant breathers are necessary for achieving maximum bearing life.
Headspace humidity control can increase bearing life by up to 40%.
Similar effects can be seen in most, if not all, wet-sump applications. Table 1 presents a comparison of water contamination levels in two different sets of splash-lubricated gearboxes: the first with active headspace humidity protection, and the second with no active headspace protection.
Both sets of gearboxes were operating in high-humidity environments outside. As can be seen from the data, protecting gearboxes with active headspace protection reduces the amount of water in the oil by up to 80%. This is an important consideration since many oils are readily hydroscopic and will absorb moisture into the free or dissolved phase if left unchecked.
Mush is made of solid particle contamination, and rightly so – particles are the leading cause of lubrication-related failure. However, do not overlook the importance of controlling moisture. For wet-sump applications, such as pumps and gearboxes, moisture is just as likely to induce failure.
Whether it’s corrosion, loss of film strength, or cavitation, water can and does have a significant impact on rotating equipment. Therefore, ensure that you take every step possible to protect your oil and equipment from the impact of free and emulsified water.