One of the first theoretical lessons you learn when you dive into the world of lubrication and lubricant analysis is that temperature generally harms these compounds. This is true, and many trainers in the area have at least a few slides dedicated to this point in their presentations.
To further elaborate on the subject, the certifying bodies also include questions about the effect of temperature on lubricants and even refer to Arrhenius’ law, a mathematical expression used to check the dependence of the speed constant of a chemical reaction on the temperature at which that reaction takes place, described by the Swedish scientist Svante Arrhenius in 1889.
The most widely spread myth about the effect of temperature on lubricant based on the Arrhenius rule is that for every 10 degrees Celsius increase, the life of the lubricant is cut in half. To what extent is this true, and to what extent is it an echo of a past when the chemistry of lubricants was very different from today?
Of course, as do operating conditions, the operating environment, and previous storage, chemistry plays an important role. Therefore, to generalize a concept to the wide range of lubricants in service would be to assume that they are all the same, which is far from reality.
Within the industrial oils market, consumption is distributed, depending on the source consulted, as follows:
- Hydraulic oils: 35-40%
- Gear oils: 20-25%
- Compressor oils: 10-15%
- Turbine oils: 5-10%
These oils work at different temperatures depending on their application; the most common are:
- Hydraulic oils: The average operating temperature is between 45°C and 65°C. In well-designed hydraulic systems, the temperature is prevented from exceeding 80°C to avoid oil degradation and ensure oil performance.
- Gear oils typically work between 60°C and 90°C, although they can reach up to 100°C under high load or speed conditions. A good system design should keep the temperature below 100°C to prevent the lubricant from oxidizing.
- Compressor oils: The operating temperature varies depending on the type of compressor. Air compressors can be between 85°C and 100°C, while refrigeration compressors can be lower, around 50°C.
- Turbine oils: They usually operate from 50°C to 60°C. Although they can easily reach 90 °C in hot spots, this is only for very short periods of time.
On the other hand, another critical piece of information that is related to the consumption and service of these oils is the replacement rate of oils in the plant, which, in its simplest form, is calculated based on the following formula:
ε: Oil substitution or replacement rate (dimensionless)
Vs: Oil volume in service
Vk: Volume of oil stored
Rf: Replacement by year or management, which is ƒ operating conditions, the type of lubricant, operating environment, and the type of lubricated component
The lower the ε, the higher the cost related to replacing the oil. This calculation allows inferring many conclusions when an audit of the plant is carried out. In my last audit of a production center with its power generation capacity, the typical values were as follows:
But let’s return to the subject of temperature. Of the oils mentioned above, the gear oils exert the greatest mechanical and temperature effort, and this article focuses on this set.
The temperature of the gears in this plant varies depending on production and time of year between 75°C and 87°C. The hypothesis raised is simple: does the operating temperature of oil have the expected effect based on the Arrhenius rule? In other words, does the temperature increase reduce the oil’s life?
The in-service gear oil is classified as an ISO VG 320 with the following characteristics.
- Synthetic base
- ISO L-CKD (ISO 12925)
- New oil viscosity of 356 cSt
- Viscosity Index 181
- AN 0.99 mg KOH/g
- Phosphorus additive 373 ppm
However, the main characteristics of this oil are easily identifiable by the infrared spectrum.
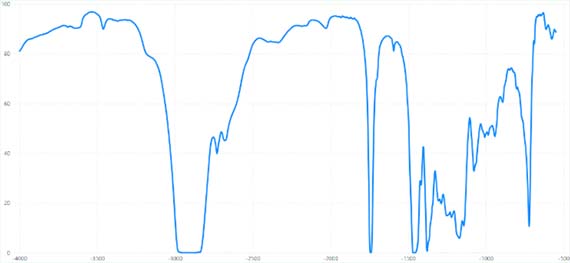
Figure 1: FTIR New Oil
The FTIR identifies, for example, the antifoaming characteristics (of the base, not the additives), the organic antioxidants such as amines and phenols, esters, and other compounds, that will be the ones on which this analysis was carried out since the changes in these are precise, easily identifiable and, above all, an early indicator of the changes undergone by the lubricant that could have an effect of reducing its expected life.
Initial Results
The oil is subjected to the same environmental and operating conditions but at a temperature of 120°C or 248°F, and samples are taken over periods of time based on a frequency established based on the estimated service life.
The most important part of this process is determining the variable or set of variables that best indicate the remaining life or loss of lubricant characteristics that show a reduction in service life.
The variables most commonly applied by any commercial lab are viscosity, acid number, additives, and oxidation.
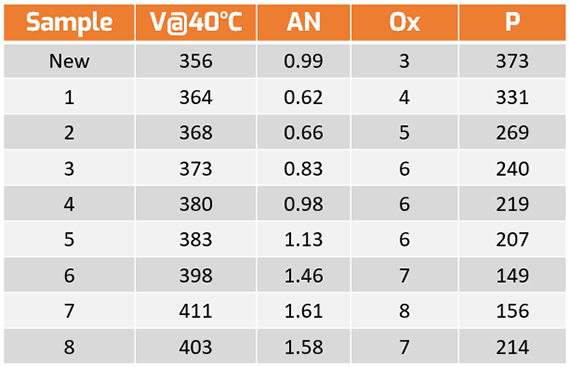
Table 1: JAB Life Reduction Analysis
Which variable or variables in the table above should be used to determine if the life of the oil has been reduced by a percentage after being subjected to a temperature increase?
There are different points of view on which we should choose one over the rest. For example, the viscosity in sample five is 7.5% above and very close to the gears’ design limit. However, in this same sample, the AN barely exceeds 14%, oxidation is in a safe zone, and additives measured as phosphorus are 55%.
Except for viscosity, these results are lagging indicators of the lubricant’s condition since the main indicators are those that actually change and subsequently give rise to those in Table 1 in compounds measured using the infrared spectrum.
Why Test These Compounds?
In this case, there are no longer just four variables to consider; we are talking about twenty different types of compounds present in this oil that can be characterized, measured, and subsequently determined by their impact on reducing the oil’s service life.
These compounds tend to change and give rise to new compounds, which can be harmful in many cases. For example, carboxylic acids, when they reach a certain concentration, can be measured indirectly logically by AN or oxidation.
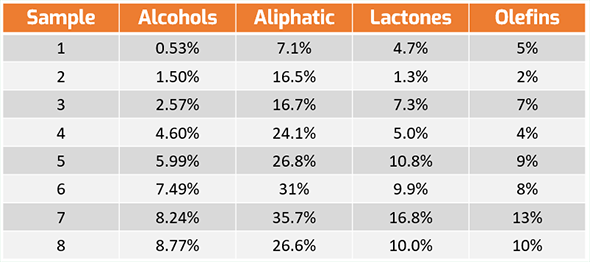
Table 2: JAB Life Reduction Analysis
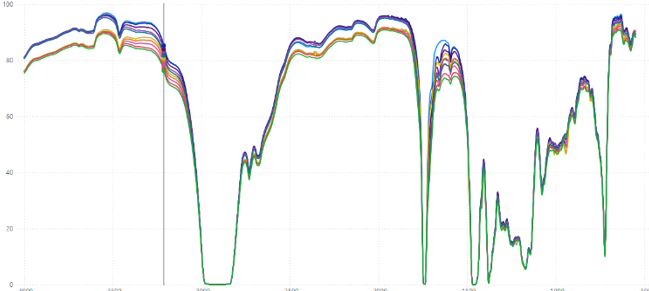
Figure 2: FTIR samples analyzed
Alcohols
Degradation-derived alcohols in industrial lubricating oils are organic compounds containing one or more hydroxyl (-OH) groups bonded to carbon atoms. These alcohols are formed as intermediates during the oxidation and thermal degradation of lubricating oil under extreme operating conditions, such as high temperatures, the presence of oxygen, and mechanical stress.
Lactones
Lactones in industrial lubricating oils are chemical compounds formed as byproducts during oil degradation and oxidation. They are cyclic esters derived from carboxylic acids and alcohols. In the context of lubricating oils, lactones are mainly generated when the hydrocarbons present in the oil are oxidized, and closed-ring structures are formed.
- Effect of lactones on oils: The presence of lactones is an indicator of advanced oil oxidation. These compounds can contribute to the oil’s acidity and negatively affect its lubricating properties, which can lead to the formation of sludge, varnishes, and corrosion on metal surfaces.
Aliphatic Acids
Aliphatic acids in industrial lubricating oils are organic compounds made up of linear or branched hydrocarbon chains that contain a carboxyl (-COOH) functional group.
- Formation of aliphatic acids in lubricating oils: Lubricating oils are subjected to extreme conditions (temperature, pressure, and exposure to oxygen) that cause the breakdown of the hydrocarbon molecules present in the oil base. This degradation leads to the formation of oxygenated compounds, such as aldehydes and ketones, which can subsequently react to form carboxylic acids, including aliphatic acids.
- Effect of aliphatic acids on oils: Increased acidity. The formation of aliphatic acids contributes to the increase in the lubricating oil’s total acid number (AN). A high AN indicates that the oil has oxidized and degraded, which can lead to corrosion of metal surfaces and negatively affect the oil’s lubricating properties.
- Wear and corrosion: Aliphatic acids can be corrosive to the metal components of the lubricated system, accelerating wear and damage to the equipment.
Olefins
Olefins are unsaturated hydrocarbons formed when oil molecules break down due to extreme temperature conditions and mechanical stress, a byproduct of thermal degradation in industrial lubricating oils.
- How olefins are formed during thermal degradation: When lubricating oil is exposed to high temperatures, the chemical bonds of the hydrocarbon molecules (which form the base of the oil) begin to break. This process is known as “thermal cracking” and results in the fragmentation of longer hydrocarbon chains.
During thermal cracking, molecular fragments containing carbon-carbon double bonds (C=C) are generated, resulting in the formation of olefins (alkenes). Due to their double bonds, these compounds are more reactive, which can lead to more side reactions, such as deposit and sludge formation.
- Effects of olefins as degradation byproducts: Increased oxidation: The olefins generated are chemically more reactive and prone to oxidation, which can lead to the formation of additional oxidation products, such as acids, varnishes, and sludge. These oxidation byproducts can damage metal surfaces and negatively affect oil performance.
Deterioration of oil properties: The presence of olefins and other thermal degradation products can change viscosity, decrease thermal and oxidative stability, and affect the oil’s lubricating properties.
Excellent Information. As An End User, When Do I Change the Oil?
It’s the million-dollar question! Let’s take it one step at a time.
First, what does an end-user in the maintenance area who struggles every day with spare parts, work planning, scheduling, orders, etc., do with this purely chemical information?
One of the most common questions in maintenance is precisely when to change the oil. Based on the analyses shown above, this same question was asked to 3 commercial laboratories.
Two of them agreed that the oil should be changed after the analysis of sample 5 based on the sample viscosity result. Most service providers have a +/- 10% limit for viscosity.
The third lab did it in sample four, based on the loss of phosphorus additive.
Draw your own conclusions from these recommendations.
Second, is traditional oil analysis useful? Of course! And a lot. The problem is that neither end users nor service providers are willing to spend time identifying that red line at which oil should be changed because its properties have been compromised or because degradation from that point will become exponential.
As has been observed, finding the lubricant failure mode is not easy, but it cannot go unnoticed. Otherwise, your lubricant condition monitoring program has not reached the degree of maturity it should and is not contributing to the maximization of the reliability and availability of plant assets.
From the third sample onwards, the degradation problem tends to become a non-linear growth trending to exponential.
The primary or early indicators of the thermal degradation reaction are closely related to those of traditional and purely commercial analysis. However, these chemical changes occur before they can be measured by a physical change, such as viscosity, for example.
In the case of service providers who perform the AN analysis manually, the problem is much greater. Assess whether it is worth working with these methods of low analytical quality.
However, we invoked the Swede Arrhenius, and his rule applied to lubrication, which must be taken with a grain of salt. We will have to see if we can apply it without putting data on the game table. Does the increase in temperature reduce the life of the oil?
In this case, you can check the characteristics of this gear lubricant above for this type of oil. The rule is not fully enforced. The temperature has increased 3 times 10°C, and even so, the oil was able to reach up to 35% above what was expected, and if we leave it in the hands of the service providers, it could reach 46%.
In a similar case, but for turbine oils, the results were the same: the lubricant could easily work ten degrees above without showing signs of degradation or loss of its remaining life.
Can Arrhenius’ rule be applied to lubrication?
Maybe, but not in all cases, and especially in new formulations where the lubricant’s nature makes it resistant to factors such as temperature or contamination.
How should I answer this question in a lubrication certification exam?
My friend! If you have come this far, I am sure you know the answer. But I advise you to answer yes or true.
What about the analytics service provider?
Value analytical quality above all else. Look for the supplier’s intensity (industrial or fleet), their expert knowledge, and how they can help you find the failure modes of your lubricants, not just issue a simple report. Choose wisely.