Varnish is characterized as a deposit originating from the degradation of lubricating oils. These degradation byproducts are predominantly polar compounds, exhibiting inherent instability within the non-polar phase of the lubricating oil. Due to this chemical incompatibility, they precipitate out of the oil solution, forming adhesive deposits on machine components.
The presence of varnish poses several reliability challenges in mechanical systems. For instance, these deposits can adhere to the internal surfaces of valves, resulting in increased friction and a propensity for the valves to adhere to their seats.
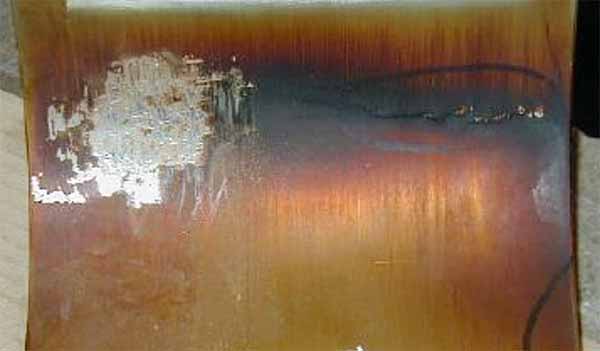
Figure 1: Deposits and severe metal wear occur in the bearing surface’s hottest zone.
This sticking phenomenon disrupts the precise control of turbines, potentially leading to erratic operation and increasing the risk of unintended shutdowns, referred to as trip events.
Additionally, the accumulation of varnish on the surfaces of heat exchangers significantly impairs their thermal efficiency. The insulating nature of these deposits hinders optimal heat transfer, thereby reducing the overall efficiency of the heat exchanger system.
Oil degradation products also tend to form on bearings, causing many deleterious operational issues. This varnish is typically hard and tenacious and can harm the bearing’s performance.
It can restrict oil flow, reduce heat dissipation, and increase friction and wear. The high temperatures of journal and thrust bearings not only accelerate oil degradation but also facilitate the hardening of these degradation products on the bearing surfaces, further accelerating deposit formation.
This article focuses on the impact of bearing deposits, the mechanisms that cause them, detection methodologies, and remediation strategies.
How Deposits Impact Bearing Performance
Oil degradation products are also well known to form on bearings, causing many deleterious operational issues. This varnish is typically hard and tenacious and can be detrimental to the bearing’s performance in three main ways:
- Act as an insulator – one of the main functions of an oil is to keep the system cool. However, with the formation of the deposits, these can act as insulators trapping heat. Therefore, the oil can no longer cool the bearing, leading to increased bearing temperature trends. This can also lead to rapid bearing temperature excursions, causing trip alarms and potentially threatening operations.
- Act as surface asperities – a bearing typically undergoes different phases of lubrication. Ideally, it should maintain a hydrodynamic film (complete separation of the two surfaces) to ensure its efficient operation. With the presence of these deposits in the high load areas, these surface asperities can interrupt the hydrodynamic film, causing the bearing to experience a mixed lubrication regime. In this condition, the bearing will have metal-to-metal contact between the two surfaces, resulting in wear or scoring, as shown in Figure 1 above.
- Induce vibrations – The mechanical impact of bearing deposits may be sufficient to cause measurable vibrations in the bearings.
Characterization of Bearing Deposits
Two distinct types of bearing deposits form through different mechanisms, each with potentially different remediation strategies.
- Cold Varnish Deposits: This category predominantly results from oil oxidation, a process where oil molecules react with oxygen, forming polar byproducts. The oxidative degradation pathway is explained in Figure 2.
These oxidation byproducts are typically aldehydes and ketones, which are quite soluble in the oil. They may undergo aggregation and agglomeration, evolving into larger molecular structures with reduced solubility in the oil.
This results in the formation of adhesive deposits. As the oil is squeezed through a bearing, it concentrates the amount of degradation products, leading to molecular cross-linking, further reducing the solubility of the degradation products, resulting in deposits.
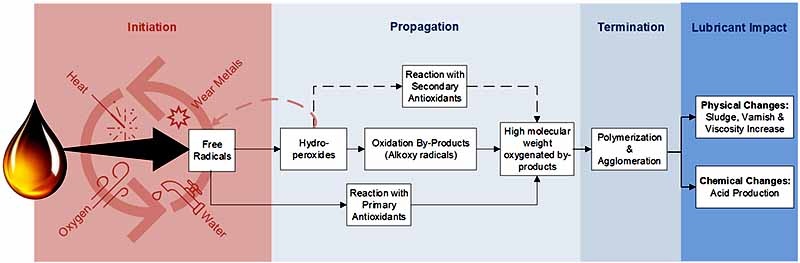
Figure 2: Summary of oil oxidation
Detection methodologies primarily include Membrane Patch Colorimetry (MPC, ASTM D7843) and Ultracentrifuge tests. These diagnostic tools are vital in providing early indicators of oil degradation presence and their potential to form deposits, enabling timely implementation of varnish removal and prevention strategies.
- Shear Stress Deposits (Hot Varnish): This deposit type is distinguished from cold varnish by its formation mechanism and chemical composition. In this case, oil degradation is driven by mechanical forces, where mechanical energy is converted into thermal energy under high shear conditions. This process results in localized temperature spikes, predominantly observed in turbomachinery operating under high-speed and heavy-load conditions.
The chemistry of these shear stress deposits differs markedly from those derived from oxidative processes as they have a higher concentration of less soluble fatty acids.
According to Chu Zhang (2017), the molecular friction generated from Shear Stress can result in temperatures reaching several hundred degrees Celsius, leading to instant degradation and the formation of localized deposits. Yulong Jiang (2021) also further explains that these high temperatures are isolated and localized in the minimum oil film thickness zone, which occurs at a molecular level.
The “Morton Effect” is a phenomenon of synchronous rotor instability due to non-uniform heating of journal bearings and is said to be caused by shear stress. The Morton Effect also causes vibration issues in turbomachinery. As per Jongh (Sep 17-20, 2018), shear stress is a dominant factor in generating non-uniform bearing temperatures.
Direct observation and temperature measurement of turbine oil during operational phases is impossible. The oil film, often only a few microns thick, precludes in-situ temperature monitoring at these scales. Nonetheless, analyzing bearing deposits can infer indirect evidence of high-temperature occurrences.
As illustrated in Figure 3 below, a bearing pad coated with varnish is examined. Samples of deposits extracted from various bearing regions underwent rigorous chemical analysis.
The findings revealed that the bulk of these deposits were organic, primarily composed of oxidized oil degradation byproducts. This chemistry contrasts with the darkest deposits, which exhibited an inorganic composition, predominantly consisting of phosphorus-based extreme pressure (EP) additives.
Under normal conditions, these EP additives remain inert, typically requiring activation temperatures around 200°C to react. The presence of these activated EP additives in the deposits strongly indicates the occurrence of localized high-temperature zones within the bearing system.
Such micro-temperature fluctuations are challenging to detect with conventional bearing thermocouple probes, underscoring the complexity of monitoring and diagnosing thermal dynamics in turbine oil films.
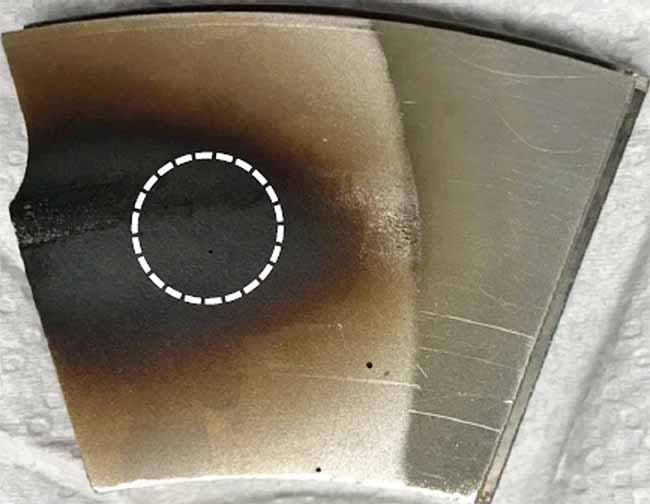
Figure 3: Bearing pad showing a dark patch of deposits at the highest load zone of the bearing. Deposit characterization of this deposit revealed that it was composed of phosphorus-based EP deposits.
Primary Drivers of Lubricant Degradation Under Shear Stress
Shear Stress Degradation is influenced by the load exerted on the bearing and its rotational speed. The critical zone of maximum load within a bearing coincides with the region where the oil film attains its minimum thickness.
As illustrated in Figure 4, empirical observations corroborate that this zone of minimal oil film thickness is the primary site for the formation of deposits.
This correlation underscores the significance of these mechanical parameters in the onset and progression of shear stress-induced lubricant degradation.
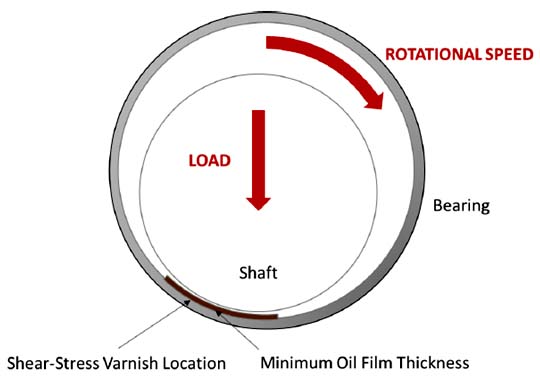
Figure 4: Illustration showing varnish formation at the minimum oil film thickness point and the two primary forces influencing Shear Stress: load and rotational speed.
It has been noted that Shear Stress deposit events occur with a greater frequency in compressors compared to turbines. This can be because the bearings in a compressor typically experience rotational speeds over 50,000 rpm.
On the other hand, the bearings in turbines usually operate at approximately 3,600rpm in the 60Hz North American market and 3,000rpm in the European and Asia Pacific markets at a frequency of 50Hz.
Detection Methods
Oxidatively derived cold varnish can be measured with the MPC or UC test as the bulk oil has experienced degradation. However, oil analysis tests are less valuable when an oil undergoes shear stress degradation.
Observing bearing temperature and vibration trends can detect shear stress deposits. Visual inspections of the bearings during outages are also valuable.
Typically, temperature spikes resembling a sawtooth-like wave pattern, as seen in Figure 5, are expected when varnish is present. A stable sawtooth waveform can also represent the presence of varnish.

Figure 5: Sawtooth bearing temperature increases
Varnish has high film strength. In some cases, the accumulation of bearing deposits can move the entire shaft. Therefore, with systems monitoring the shaft position, utilizing the gap voltage vertical probe measurement will be worthwhile.
As shown in Figure 6 below, the shaft moves away from the center position as the temperature increases due to deposit accumulation. The shaft’s vertical position shift correlates closely with a bearing temperature spike.
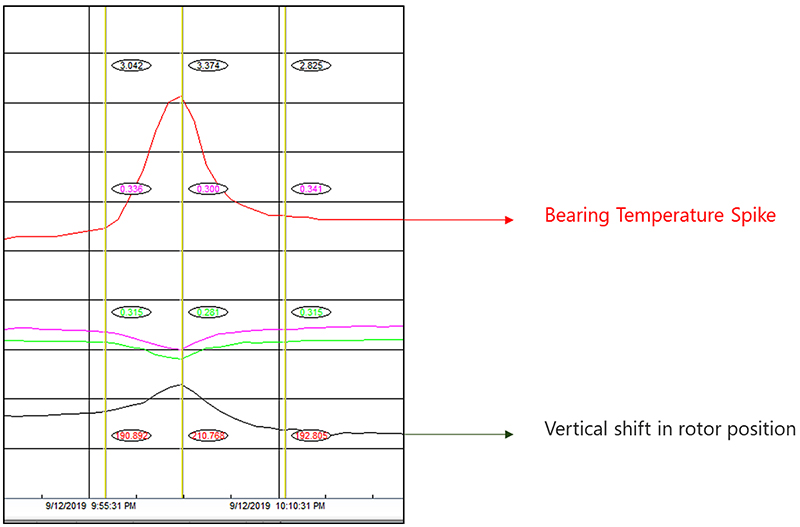
Figure 6: Bearing temperature increases as the varnish layer grows due to its insulating properties. The correlation between bearing temperature and rotor position suggests that as the varnish layer increases, the rotor also moves away from the center.
Bearing temperature excursions can cause operational disruptions. Below are some noteworthy bearing temperatures based on API 670:
- 110° C Alarm Temperature
- 120° C Trip Temperature
- 132° C Babbitt Creep
- 232° C Babbitt melting point
How can Bearing Deposits be Mitigated?
Cold Varnish
Oxidatively-derived bearing deposits can be removed by installing a resin-based filtration system, such as Electrophysical Separation Process (ESP) technology.
It can also be prevented or removed using a solubility enhancer, such as DECON. The impact of these technologies results in bearing temperature decreases, low MPC values, elimination of bearing wear, and increased efficiency due to lower friction.
Figure 7 shows an example of a bearing in a steam turbine before and after an ESP system was installed.
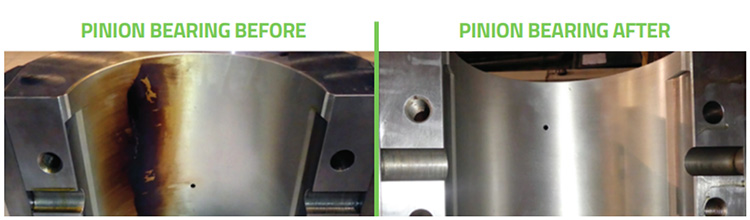
Figure 7: ESP has been shown to clean bearing deposits
Hot Varnish
Shear Stress Deposits have been observed in Group I, II, III, and IV base oil formulations. This suggests that simply changing the oil to another base stock formulation will not alleviate the issue. However, there are potential mechanical and chemical solutions.
Mechanical Solutions
From a mechanical aspect, Shear Stress Deposits can also be controlled by reducing the load on the oil. Some mechanical fixes include;
- Reducing the load (this is operationally feasible but reduces compressor output, making it a costly option)
- Expanding bearing clearances (Jongh, Sept 17-20, 2018)
- Offsetting the bearing pivot towards its lagging side has been proven to improve the angle of attack and allow more oil to flow
- Directional lubricating (Bloch, July 2006)
The Chemical Solution
Adding a solubility enhancer is a potential chemical solution to this mechanical problem. DECON has been shown to have an immediate impact on deposits and can lower the temperatures in bearings. It is an oil-soluble cleaner designed to be added at 3-5%.
It has been designed for long-term use, which can provide a permanent solution to bearing deposits. This product effectively mitigates deposit formation by enhancing the solubility of the in-service oil, enabling the dissolution of degradation byproducts.
Often, varnish products comprise depleted antioxidants, inorganic additives, and degraded hydrocarbon molecules. DECON is designed to soften the carbon-based deposits upon which the inorganic deposits (such as the aldehydes, ketones, and fatty acids found on journal bearings) usually adhere. Thus, when these carbon-based deposits soften, they can be easily removed.
When these deposits are dissolved into the oil, they remain inert and pose no operational concerns until they principate out of the solution. Dissolving these deposits back into the oil does not adversely impact the oil’s condition, nor does it pose a risk of catalyzing further degradation.
Figure 8 shows the temperature graph after adding 3% of DECON to a compressor suffering from shear-stress deposits. The temperature excursions stopped after a few hours.
Not all mechanical issues can be solved through chemistry. However, DECON is cost-effective for bearings experiencing shear stress and oxidatively derived deposits.
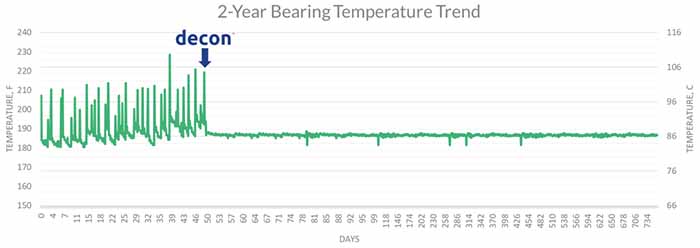
Figure 8: The impact of adding 3% DECON to the bearing temperatures of a compressor.
Summary of Differences Between Hot Varnish and Cold Varnish on Bearings
The following table summarizes the differences between hot and cold bearing deposits.
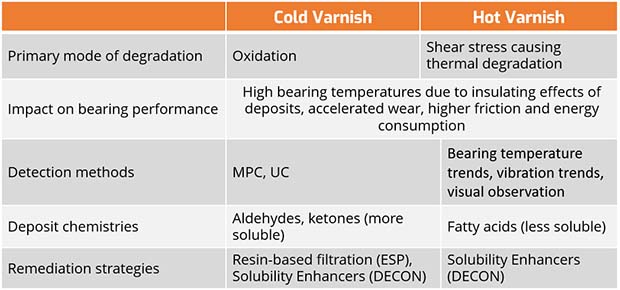
Table 1: Cold vs Hot Varnish
Summary
Bearing deposits can have a significant impact on the operation of rotating equipment. They lead to high bearing temperature excursions, wear, and increased friction. Two different types of deposits are found on bearings: oxidatively-derived cold varnish and Shear Stress-derived hot varnish.
The traditional methods of utilizing the MPC and UC (Ultracentrifuge) tests to detect the presence of cold varnish in equipment are ineffective in detecting hot varnish or shear stress deposits. By monitoring bearing temperatures and vibration, operators have an increased opportunity to detect the presence of shear stress deposits.
Bearing deposits experiencing cold varnish may be remedied by installing a resin-based filtration system, such as ESP technology, or adding a solubility enhancer, such as DECON.
The remedy to shear stress deposits is often a mechanical fix that may spread the bearing load over a greater oil surface to reduce load, especially in the minimum oil film thickness zone. Alternatively, DECON has been shown to be an effective chemical solution to shear-stress deposits.
This is article is based on the white paper ” Understanding Shear Stress and the formation of deposits in heavily loaded, high-speed bearing applications” by Greg Livingstone (Fluitec) and Cody A. Evans (Mobil).
References:
- Chu Zhang, J.-G. Y.-S. (2017). Influence of Varnish on Bearing Performance and Vibration of Rotating Machinery. International Journal of Rotating Machinery, Article ID 9131275, 10 pages.
- Jongh, F. d. (Sept 17-20, 2018). The Synchronous Rotor Instability Phenomenon – Morton Effect. 47th Turbomachinery & 34th Pump Symposia, Houston, Texas, 12.
- Yulong Jiang, B. L. (2021). Prediction on Flow and Thermal Characteristics of Ultrathin Lubricant Film of Hydrodynamic Journal Bearing. Micromachines, 12, 1208.
- Bloch, H. (July 2006). Tilting Pad Thrust Bearings. Machinery Lubrication. July 2006.